
Storage Lab
Comparative life cycle assessment of lithium-ion battery chemistries for residential storage
Residential storage deployment is expected to grow dramatically over the coming decade. Several lithium-ion chemistries are employed, but the relative environmental impacts of manufacturing them is poorly understood.
This study presents a cradle-to-gate life cycle assessment to quantify the environmental impact of five prominent lithium-ion chemistries, based on the specifications of 73 commercially-available battery modules used for residential applications. Three impact categories (global warming potential - GWP, cumulative energy demand - CED and mineral resource scarcity - MRS) are analysed across two functional units (storage capacity and lifetime energy delivered).
​
Figure 1 depicts the GWP and MRS factors by components for 8.1 kWh lithium-ion batteries across the five chemistry types commercially available. It is observed that cells only constitute a third to a half of the environmental impact, which is comparable to the balance-of-system components and assembly or the inverter. The lower capacity of NCO-LTO cells means that many more cells are required for the required system capacity than for the other technologies, resulting in higher GWP and MRS impacts. However, this effect will be balanced by the much higher cycle life and delivered lifetime energy of this chemistry.


Figure 1 - Global warming potential (left) and mineral resource scarcity (right) contribution from different components of an 8.1 kWh residential lithium-ion battery system. Higher values indicate higher ecological impact. The error bars represent the standard deviation across the distribution of performances reported by the manufacturers of battery modules of a same chemistry in our sample. BMS: Battery management system.
Figure 2 analyses the energy delivered on energy invested (EDOEI), a metric that indicates how much of the energy invested in manufacturing (and charging) the battery is delivered during its operational life. EDOEI is calculated at 2–4 and falls to 0.54–0.66 with the energy for charging included (cf. a round-trip efficiency of 82–89%).
When considering different cycling frequencies (i.e., energy delivered each day) at a given calendar lifetime of battery systems, it becomes clear that environmental impact depends more on cycling frequency than chemistry choice.
Routes to making residential lithium-ion battery systems more environmentally benign include reducing the reliance on cobalt, nickel and copper, increasing the specific usable energy, developing comprehensive recycling initiatives, and maximising the utilisation (cycle frequency) once in operation.

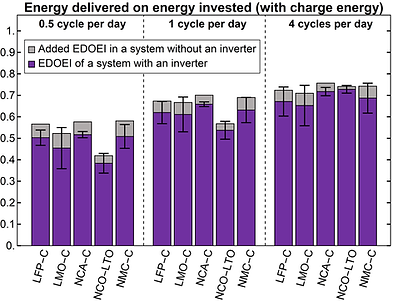
Figure 2 - The lifetime energy delivered on energy invested for different lithium-ion battery chemistries and cycling intensities, excluding (left) and including (right) the energy used for charging. This metric is based on the impact indicator for cumulative energy demand. Higher values are more energy efficient. Coloured bars represent the EDOEI of the complete battery system, grey bars show the EDOEI of the battery excluding the inverter. The error bars represent the standard deviation across the distribution of performances reported by the manufacturers of battery modules of a same chemistry in our sample.
Le Varlet, T., Schmidt, O., Gambhir, A., Few, S. & Staffell, I. Comparative life cycle assessment of lithium-ion battery chemistries for residential storage. J. Energy Storage 28, 101230 (2020). (Publication) (Accepted manuscript)